Translate this page into:
Apoptosis, DNA damage, and cell cycle arrest: The anticancer mechanisms of quercetin in human cervix epithelioid carcinoma cells
*Corresponding author: Sabiha Fatima, Department of Clinical Laboratory Sciences, College of Applied Medical Sciences, King Saud University, Riyadh, Saudi Arabia. sabmehdi@ksu.edu.sa
-
Received: ,
Accepted: ,
How to cite this article: Fatima S, Borappa M, Kanakarajan S, Selvaraj R, Siddiqi NJ, Wasi S, et al. Apoptosis, DNA damage, and cell cycle arrest: The anticancer mechanisms of quercetin in human cervix epithelioid carcinoma cells. Inter J Health Sci. 2025;19:43-51. doi: 10.25259/OA05_8747
Abstract
Objectives
The objectives of the study are to investigate the anti-cancer properties of quercetin, a widely distributed phytochemical, and assess its potential as a chemopreventive and therapeutic agent for cervical cell carcinoma.
Methods
The study employed cell viability assays for cytotoxicity, flow cytometry for cell cycle arrest, comet assays for DNA damage, nuclear morphology to assess apoptosis, and Western blotting to measure caspase 3 expression, a key apoptosis-related marker.
Results
Notably, a significantly lower half-maximal inhibitory concentration (25.5 μM) at 48 h compared to 24 h highlights the time-dependent nature of quercetin’s cytotoxic effects. Quercetin significantly (P < 0.001) inhibited human cervix epithelioid carcinoma (HeLa) cell viability compared to the control group, indicating its cytotoxic potential. Morphological changes observed in quercetin-treated cells further supported its cytotoxic effects. Quercetin-induced substantial increase in the percentage of cells in the sub-G0/G1 phase relative to controls (P < 0.001) reveals cell cycle arrest and the initiation of apoptosis. In addition, quercetin caused notable DNA damage, as evidenced by the significant increase (P < 0.001) in the comet tail length, highlighting the genotoxic effects of quercetin. Moreover, quercetin treatment significantly (P < 0.001) upregulated the expression of caspase-3, suggesting the activation of the intrinsic apoptotic pathway.
Conclusion
The findings of the current study suggest that quercetin can effectively inhibit HeLa cell viability, induce cell cycle arrest, promote DNA damage, and activate caspase-mediated apoptosis. This underscores quercetin’s therapeutic potential against cervical cancer and highlights caspase-mediated apoptosis as a promising strategy. Prolonged exposure may further enhance its efficacy, offering valuable insights into its potential for long-term cancer treatment.
Keywords
Apoptosis
Caspase-3
Cell cycle arrest
Cytotoxicity
Human cervix epithelioid carcinoma cells
Quercetin
INTRODUCTION
Cancer is a major contributor to global deaths, and the treatments often cause serious side effects, making life difficult for patients. Globally, cervical cancer is the fourth most prevalent cancer in women. The incidence rate is higher in middle and low-income countries and lower socio-economic strata within countries.[1,2] Human papillomavirus (HPV) is the most common cause of cervical cancer, with 90% of cases exhibiting viral DNA from a particular subset of HPV.[3,4] Hence, there is a growing interest in shifting away from aggressive chemotherapy toward more precise and mechanism-driven prevention approaches. Research consistently shows that eating a diet rich in fruit and vegetables may lower cancer risk and the likelihood of mortality associated with the disease.[5,6]
Plants and their derivatives are rich in phytochemicals with antioxidant, anti-inflammatory, and pro-apoptotic properties.[7,8] These compounds, including flavonoids, isoflavonoids, lignans, polyphenols, and terpenoids, play a key role in cancer prevention by modulating cell signaling pathways and promoting apoptosis.[9,10] They have garnered attention for their potential in populations with low incidences of cancers such as colon, breast, liver, and prostate.[5,6,11-15] However, the mechanisms through which phytochemicals influence cancer progression and cell death require further exploration to understand their pharmacological and cellular effects.
Quercetin is a naturally occurring flavonoid found in various fruits, vegetables, and plants, including apples, onions, berries, citrus fruits, grapes, broccoli, and tea. It is also abundant in capers and herbs such as dill and cilantro. Pharmacologically, quercetin is known for its antioxidant, anti-inflammatory, anticancer, and antiviral properties.[16] However, its bioavailability is relatively low due to poor water solubility and rapid metabolism, which limits its therapeutic effectiveness. The therapeutic dose varies depending on the condition but typically ranges between 500 and 1000 mg/day in clinical settings.[17,18] Studies conducted both in vitro and in vivo have revealed that quercetin exhibits properties that inhibit tumor growth and promote apoptosis, affecting several types of tumors, such as those in the stomach, colon, breast, and ovary.[19] Quercetin has been investigated in only a few clinical trials for its anticancer effects in the past decade.[20,21] As per the data on ClinicalTrials.gov., numerous current studies have investigated quercetin’s anticancer properties. However, while many trials are marked as completed, their results are still unavailable. Thus, comprehensive clinical evidence supporting quercetin’s efficacy in cancer treatment remains limited.
Despite quercetin’s promising potential in inhibiting cell proliferation, its exact mechanism is still poorly understood. This knowledge gap prompted the present study, which aims to investigate the apoptotic effects of quercetin and elucidate its underlying mechanisms in human cervix epithelioid carcinoma (HeLa) cells. By employing a combination of assays, including cell viability, flow cytometry, nuclear morphology assessments, comet assays, and western blotting, this research aims to establish a clearer connection between quercetin treatment and apoptosis. Furthermore, determining the half-maximal inhibitory concentration (IC50) value of quercetin across different exposure periods will offer valuable insights into its therapeutic window and effectiveness. This study will address critical gaps in understanding quercetin’s mechanisms, thereby contributing to the development of more targeted pharmacological and clinical approaches for cancer treatment.
MATERIALS AND METHODS
The quercetin compound (Cat. No. - Q4951) was obtained from Sigma Aldrich, India. HeLa cells were procured from the National Centre for Cell Science in Pune, India.
Culturing HeLa cells
The cells were cultured in T-25 culture flasks containing Dulbecco’s Modified Eagle’s Medium (DMEM) supplemented with 10% fetal bovine serum (FBS), penicillin-streptomycin, and sodium bicarbonate. The flasks were incubated at 37°C in a humidified atmosphere with 5% carbon dioxide until reaching 70–80% confluency.
Standardization of quercetin by 3-(4,5-dimethylthiazol-2-yl)-2,5-diphenyl-2H-tetrazolium bromide (MTT) assay
HeLa cells at a density of 1 × 104 cells/mL were seeded in 96-well plates and incubated for 24 h. Following attachment, the cells were exposed to a range of quercetin concentrations (10, 25, 50, 75, and 100 μM), with subsequent incubation for 24 and 48 h.
Vehicle control cells were treated in a similar manner using 0.1% dimethyl sulfoxide (DMSO). The morphological changes of quercetin-treated HeLa cell lines were observed using inverted microscopy. After treatment, in each well MTT (sigma-Aldrich, India) solution (0.5 mg/mL) was added and incubated for 3 h. Following incubation, DMSO was added and incubated for 20 min to dissolve the formazan crystals. The absorbance was then measured at 570 nm, and cell viability was calculated using the standard formula. The IC50 concentration was subsequently determined.
Nuclear staining and apoptotic morphology (Annexin V-fluorescein isothiocyanate [FITC]/propidium iodide [PI] staining)
To analyze apoptotic cell death, the nuclear morphology of HeLa cells was visualized under a fluorescent microscope using filters appropriate for Annexin V-FITC/PI stain. For attachment, 1 × 106/HeLa cells per well were added in six-well plates and incubated overnight. This was followed by treating the cells for 48 h with an IC50 concentration of quercetin. The harvested cells were washed with phosphate buffer saline (PBS) twice and re-suspended in ×1 binding buffer (500 μL). The cells were incubated in the dark at room temperature for 15 min. Subsequently, they were stained with Annexin V-FITC (5.0 μL) conjugate and 10.0 μL of PI before being observed under a fluorescent microscope.
Assessment of DNA damage by comet assay
The HeLa cells were exposed to IC50 48 h concentration and maximum concentration of quercetin for 48 h along with control cells. The control cells were treated with 0.1% DMSO. The DNA from the cells was harvested using agarose gel electrophoresis. Using a horizontal electrophoresis system, the electrophoresis of the slides was carried out. After gently removing the slides from the lysis solution, they were placed in the electrophoresis tank filled with buffer. After allowing DNA to unwind in the buffer for 20 min, the slides were electrophoresed at 0.8 Volts/cm for 15 min. Next, the slides underwent three rinses in a neutralization buffer before being dried. A PI working solution drops were added to the gel and a cover slip was placed over it. The stained DNA was examined with magnifications using a fluorescent microscope. Comet Assay Software Project (CASP) software was used to measure the length of migrated DNA (Comet tail).
Examination of cell cycle arrest through flow cytometry
Flow cytometric analysis was conducted to measure the phases of cell cycle distribution. For synchronization in the G0/G1 phase, HeLa cells were cultured in serum-free DMEM for 36 h. The medium was then replaced with 10% serum-supplemented medium with 0.1% DMSO (control), and quercetin (at its 48-h IC50 concentration) was added to the appropriate wells for 48 h. The floating cells were gathered with the attached cells in the medium and then harvested by trypsinization. The cells were washed with cold PBS, fixed in ethanol (80% in PBS) at −20°C, and centrifuged. The resulting pellets were stained for 30 min with PI (50 μg/mL) and RNase A (20 μg/mL) at 37°C. Approximately ≥20,000 cells were analyzed using a flow cytometer. The analysis of cell cycle histograms was performed using “Cell Quest” software.
Western blot analysis
The expression levels of Caspase-3 protein, a final executor of apoptosis, and β-Actin used as a control were evaluated in untreated control HeLa cells and those treated with quercetin at its IC50 concentration for 48 h. A mixture of 50 mg of cell lysates and ×2 sample buffer was boiled for 5 min. Afterward, the mixture was transferred onto a polyvinylidene fluoride (PVDF) membrane following 2-h electrophoresis on 12% Sodium dodecyl sulfatepolyacrylamide gel electrophoresis (SDS-PAGE) gels at 100 V. Subsequently, membranes were blocked overnight with 10% non-fat dried milk blocking buffer and then incubated with primary antibodies against Caspase-3 (1:1000; sc-7272) and β-Actin (1:1000; sc-47778) from Santa Cruz Biotechnology, Inc., Dallas, TX, USA for 6 h. After washing, membranes underwent incubation with a horseradish peroxidase-linked secondary antibody. Protein expression was detected using 3,3’-diaminobenzidine (DAB) (Thermo Fisher Scientific Inc., MA, USA), and band intensities were quantified with a GS-670 imaging densitometer (Bio-Rad, Hercules, CA, USA). The expression levels of Caspase-3 were normalized to β-Actin, serving as the control.
Statistical analysis
The results are expressed as the mean ± standard error from five individual data points. The statistical significance of the sample was evaluated using “two-way analysis of variance” through Prism GraphPad 5 software, with a significance threshold set at P < 0.05.
RESULTS
Quercetin-induced inhibition of HeLa cell viability
Quercetin was tested for its impact on HeLa cell viability by exposing the cells to varying concentrations and measuring the results with an MTT assay [Figure 1]. HeLa cells were exposed to increasing concentrations of quercetin (10, 25, 50, 75, and 100 μM) for 24 and 48 h. Quercetin treatment resulted in significant growth inhibition of HeLa cells, which was both time and dose-dependent. Treatment with 10 μM quercetin caused a reduction in HeLa cell viability by 11.5% after 24 h and 23.4% after 48 h. In contrast, a 25 μM concentration of quercetin decreased cell viability by approximately 22.6% at 24 h and 48.2% at 48 h [Figure 1]. The effective concentration for 50% (IC50) inhibition of cell viability was determined to be 96.24 μM at 24 h and 25.5 μM at 48 h. Based on these preliminary viability studies, a concentration of 25.5 μM (the IC50 for 48 h) was selected for subsequent experiments.
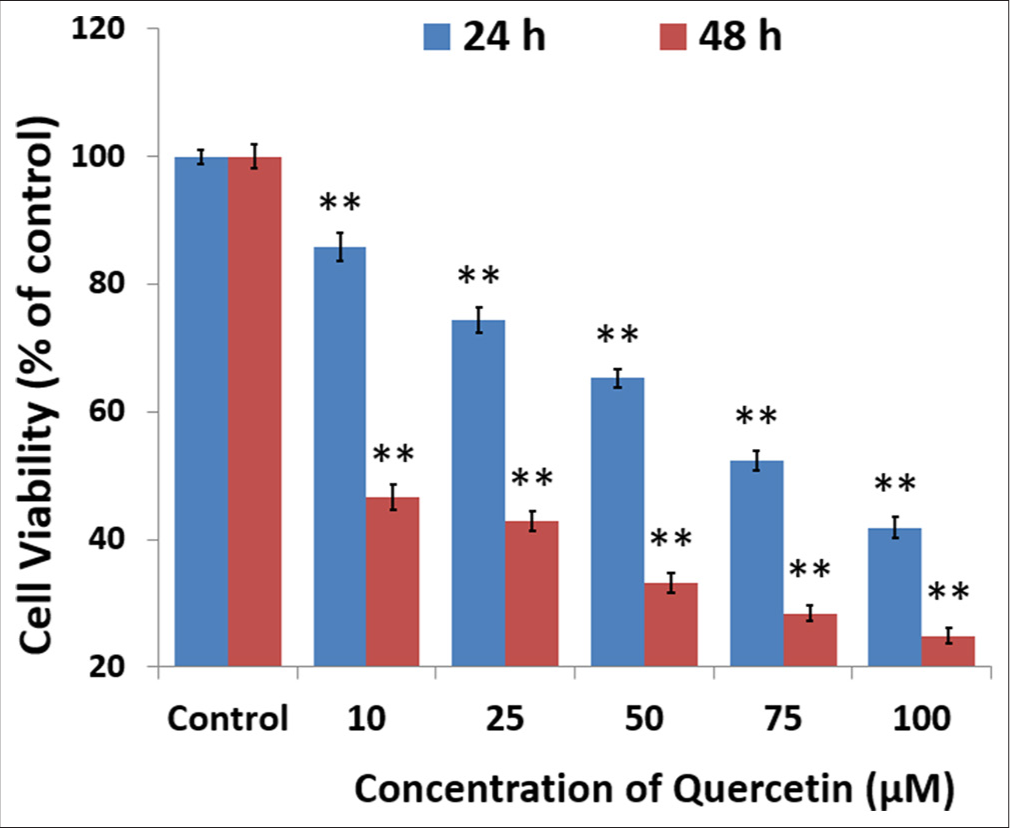
- Quercetin decreased the viability of human cervix epithelioid carcinoma cells. The cells were exposed to quercetin at 10, 25, 50, 75, and 100 μM concentrations for 24 and 48 h, using dimethyl sulfoxide as the solvent control. The 3-(4,5-dimethylthiazol-2-yl)-2,5-diphenyl-2H-tetrazolium bromide cell viability assay demonstrated a reduction in cell survival that depended on both dose and time, with results observed at 24 h (blue) and 48 h (red). The half-maximal inhibitory concentration values of quercetin were determined to be 95.5 μM at 24 h and 25.5 μM at 48 h. Values are mean ± standard error of six individual observations. ** indicate significance at P < 0.001 relative to control.
Cytomorphological changes in quercetin-treated HeLa cells
The cells treated with the IC50 concentration of quercetin for 48 h showed a clear change in cell morphology indicative of unhealthy cells. In contrast, control cells retained their characteristic polygonal and elongated appearance [Figure 2]. The quercetin-treated cells showed a round shape with apoptotic body formation, indicating typical features of cells undergoing apoptosis. These results strongly suggest that quercetin induces alterations in cell morphology, which in turn causes a decrease in cell viability.
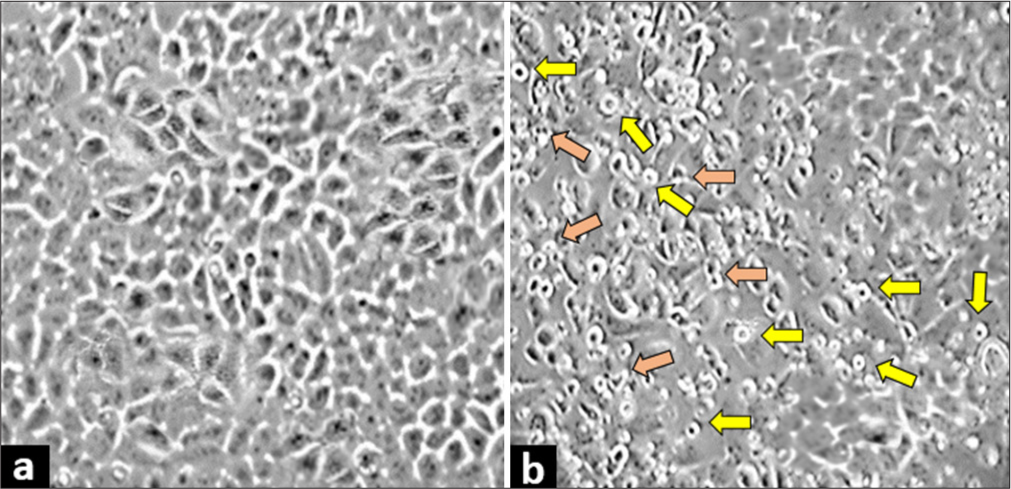
- Quercetin treatment induced morphological changes in human cervix epithelioid carcinoma cells. Morphology of (a) untreated control cells and (b) cells treated with half-maximal inhibitory concentration of quercetin. The cells exhibited morphological changes, such as a round shape (yellow arrows), and forming apoptotic bodies (orange arrows), which is a sign of cell death. These changes were observed at ×400 magnification under a light microscope.
Quercetin-induced cell cycle arrest in HeLa cells
Apoptosis induces cell death in proliferating cancer cells either by inhibiting a specific phase of the cell cycle or by damaging DNA extensively, resulting in DNA fragmentation. To explore this, cell cycle distribution was performed using flow cytometry to investigate its association with reduced viability. Quercetin treatment showed a significant (P < 0.001) increase in the number of cells gated in the sub-G0/G1 phase compared to untreated cells [Figure 3]. This indicates that the quercetin at the IC50 concentration for 48 h causes cell cycle arrest in the sub-G0/G1 phase.
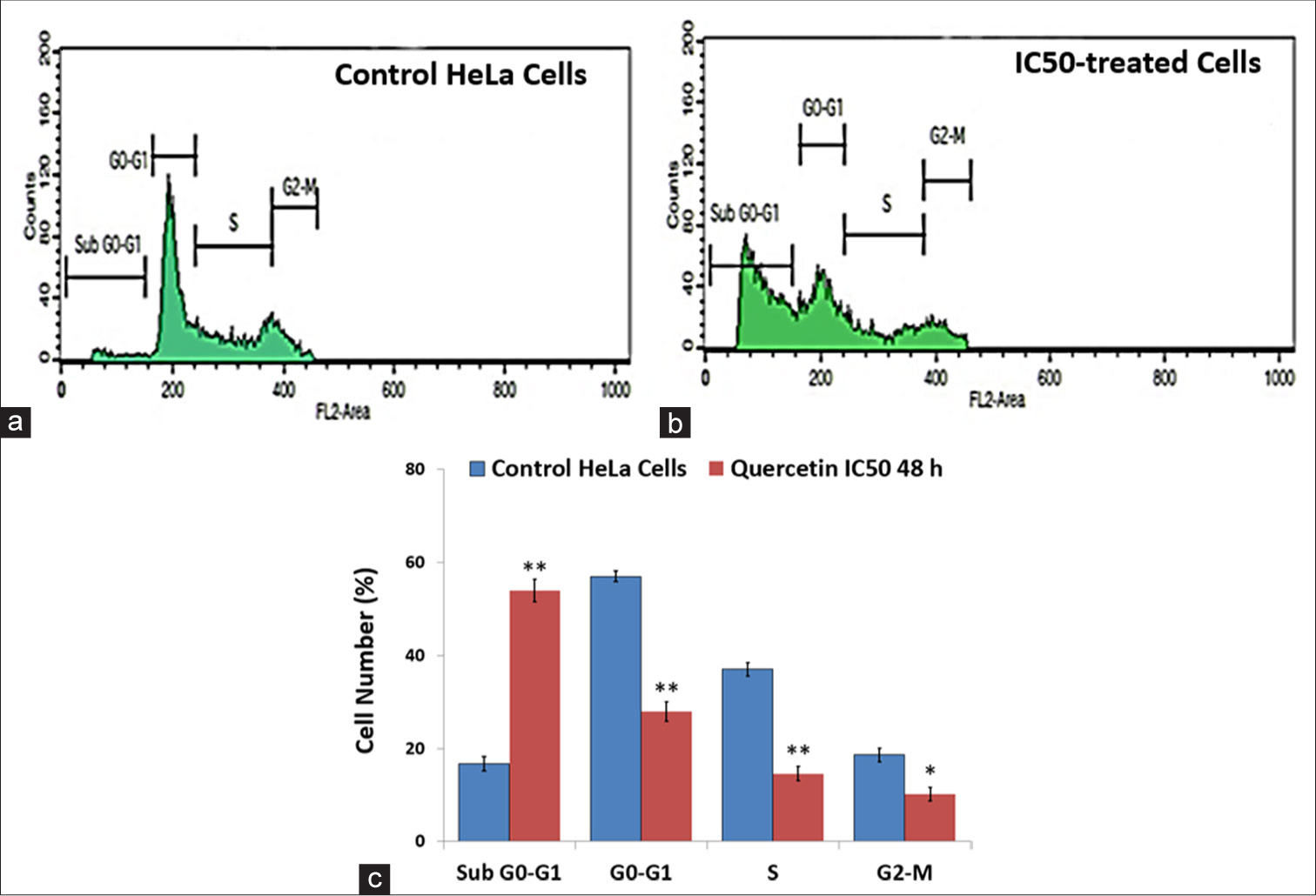
- Flow cytometry was used to analyze the cell cycle distribution. (a) Untreated control human cervix epithelioid carcinoma (HeLa) cells and (b) HeLa cells exposed to quercetin exhibited a significant rise in the proportion of cells accumulating in the sub-G0-G1 phase. (c) A bar graph illustrates the distribution of HeLa cells across different cell cycle phases following 48 h of treatment with quercetin. * indicate significance at P < 0.01 relative to control, ** indicate significance at P < 0.001 relative to control.
Quercetin-induced nuclear morphological changes and apoptotic body formation in HeLa cells
Annexin V-FITC and PI staining were used to assess the effect of quercetin on the nuclear morphological changes as a sign of apoptosis and a cause of reduced cell viability. The cells were observed and photographed under a confocal microscope The cells treated with IC50 concentration of quercetin at 48 h showed increased nuclear fragmentation and nuclear condensation with apoptotic body formation compared to the untreated control HeLa cells [Figure 4a and b]. Therefore, it can be concluded that quercetin treatment resulted in the induction of cell death.
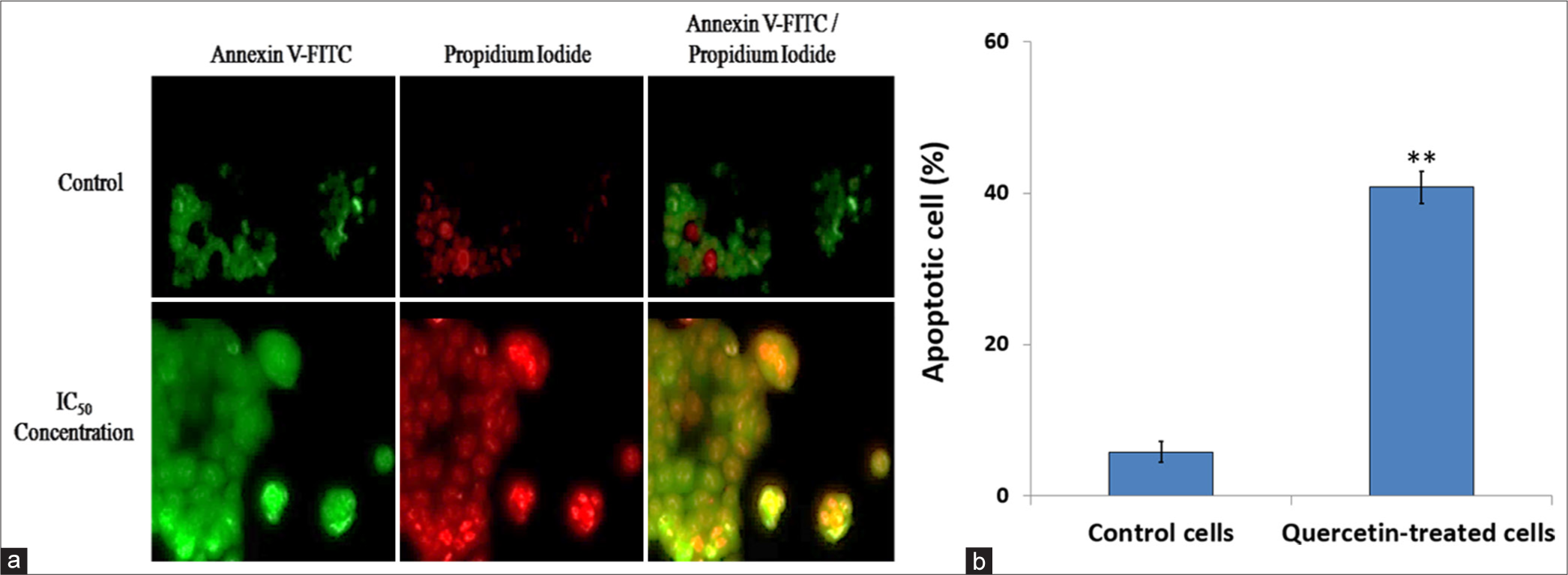
- Apoptosis was evaluated through staining with Annexin-V fluorescein isothiocyanate (FITC) and propidium iodide. (a) The images show apoptotic cells (green), necrotic cells (red), and late apoptosis stages (merged) in human cervix epithelioid carcinoma (HeLa) cells treated with quercetin at the 48-h half-maximal inhibitory concentration (IC50), compared to untreated control cells. When statistically analyzed, (b) quercetin-treated HeLa cells demonstrated a significantly higher apoptosis rate than control HeLa cells. ** indicate significance at P < 0.001 relative to control.
Quercetin-induced DNA damage in HeLa cells evidenced by comet assay
Arrest in the sub-G0/G1 phase of the cell cycle typically indicates apoptotic cells with DNA damage. To assess the effect of quercetin on DNA damage, an alkaline comet assay was conducted on HeLa cells exposed to quercetin at its IC50 concentration for 48 h. The control cells showed no signs of DNA fragmentation. The cells treated with quercetin-induced DNA damage manifested as a comet-like tail in HeLa cells. The untreated cells exhibited negligible DNA damage [Figure 5a], while quercetin treatment caused significant (P < 0.001) DNA damage indicated by comet-like tail cells moving out of the nucleus [Figure 5b]. The length of the comet tails was quantified and is shown in the graph in Figure 5c.
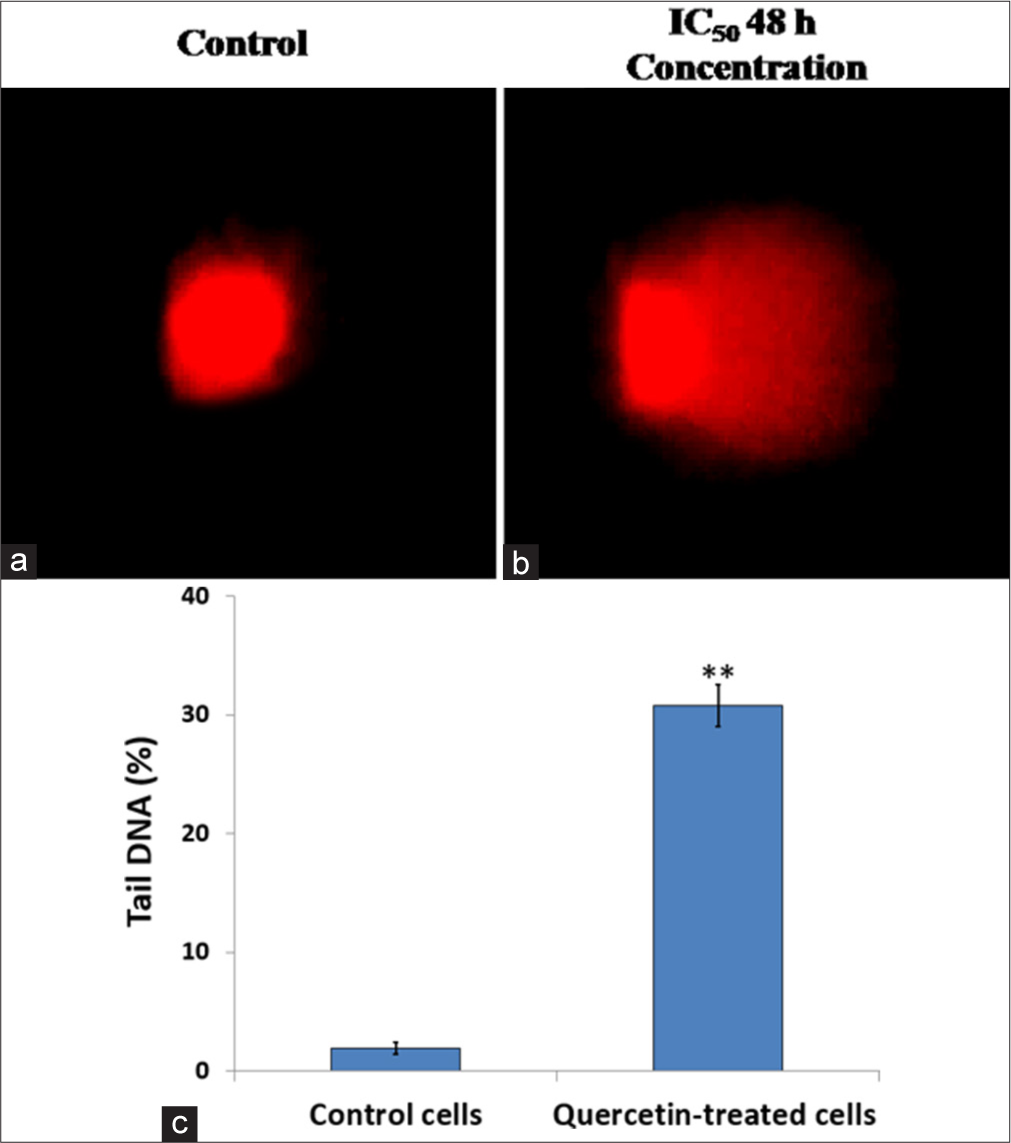
- DNA damage was assessed using the comet assay. (a) Control human cervix epithelioid carcinoma (HeLa) cells and (b) HeLa cells treated with quercetin at the half-maximal inhibitory concentration (IC50) for 48 h were examined. (c) Compared to untreated HeLa cells, a quantitative analysis of comet assay results showed a quercetin-induced increase in DNA damage. ** indicate significance at P < 0.001 relative to control.
Quercetin-induced caspase-3 activation
We observed that quercetin-induced characteristic morphological and biochemical changes associated with apoptosis, such as DNA damage in HeLa cells. Hence, we further assessed the expression of caspase 3, the executioner caspase, which is involved in the final stages of apoptosis and responsible for many biochemical changes that lead to cell death. Figure 6 shows the effect of quercetin treatment on the protein expression of Caspase-3, with β-actin serving as the control. Treatment of HeLa cells with IC50 concentration of quercetin caused a dose-dependent increase in the level of active fragments of Caspase-3 (17 kDa) significantly (P < 0.001) when compared to the untreated control, indicating the activation of Caspase-3 after quercetin treatment.
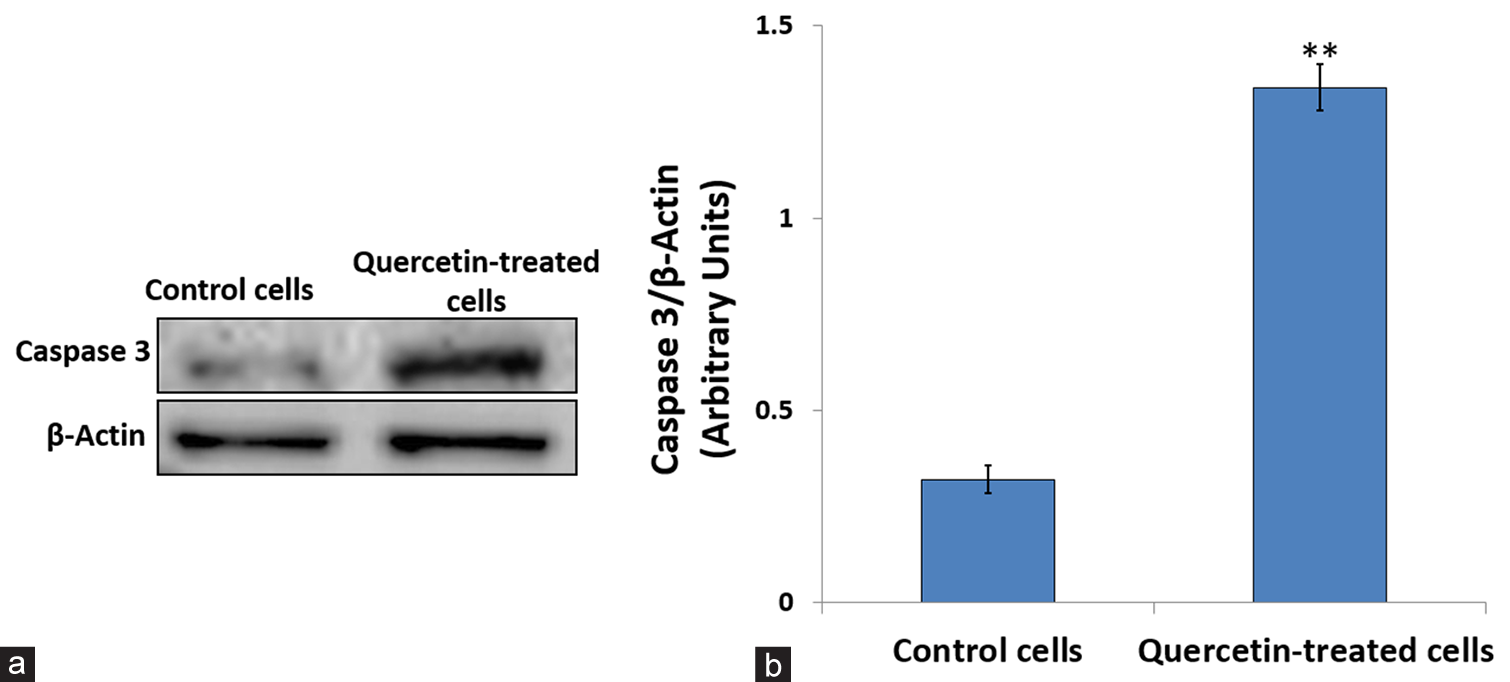
- Effect of 48 h of quercetin treatment on caspase-3 expression. (a) Western blot bands showing caspase-3 protein levels in untreated and quercetin-treated human cervix epithelioid carcinoma cells. (b) The caspase 3 expression level was quantified by comparing it to β-Actin, an internal control. ** Indicate significance at P < 0.001 relative to control.
DISCUSSION
The prevalence and mortality of cancer have been rising globally despite significant advances in our understanding and treatment of the disease. This has led to increased interest in chemoprevention using natural dietary compounds. Therefore, natural products are attractive candidates for developing anticancer drugs. Quercetin, a secondary metabolite found ubiquitously in various plant parts, is a powerful antioxidant known for inhibiting lipid peroxidation and enhancing antioxidant enzyme activities.[22] It has been reported to inhibit UV-B-induced damage in HaCaT cells.[23] Many in vivo and in vitro investigations have confirmed the ability of quercetin to inhibit malignant growth and metastasis in different cell types. Quercetin’s anticancer mechanism involves cellular cytotoxicity, modulation of signaling pathways, apoptosis induction, metastasis suppression, and drug resistance reversal.[24] In this study, we examined quercetin’s effects on HeLa cells, focusing on its cellular cytotoxicity and pro-apoptotic properties. Compared to other studies, our study demonstrates a stronger anti-cancer effect of quercetin on HeLa cells at 48 h. Previous studies have reported that a higher IC50 concentration, such as 110.38 μM for 18 h and 100 μM for 24 h,[25,26] aligns closely with IC50 concentration (96.24 μM) observed in our study at 24 h [Figure 1]. The lower IC50 of 25.5 μM at 48 h suggests that quercetin may become more effective over time, leading to greater cytotoxicity with prolonged exposure.
Quercetin is a commonly occurring flavonoid that has been shown to inhibit key events of carcinogenesis, such as proliferation and migration of cancer cells.[27] Our flow cytometry results demonstrated that quercetin-induced Sub G0/G1 phase arrest [Figure 3], which is in contrast with previous studies showing G2-M arrest in HeLa, human bladder cancer, esophageal adenocarcinoma, and breast carcinoma.[26,28-30] Quercetin has been found to cause the induction of apoptosis and cell cycle arrest in the G2/M phase in MCF-7 cells.[28] In the NALM6 leukemic cell line, increasing doses of quercetin led to an accumulation of sub-G0 apoptotic cells, whereas lower concentrations caused S-phase arrest.[31] This indicates that different cell types can exhibit varying responses to quercetin. To further assess the apoptosis induced by quercetin in HeLa cells, Annexin-V and PI staining and comet assays were conducted. Apoptosis in quercetin-treated HeLa cells was significantly (P > 0.001) high as compared to control cells [Figure 4]. The finding was consistent with the morphological changes, and induction of apoptosis was further supported by the comet assay as apoptosis can be triggered by DNA damage. Quercetin treatment with IC50 concentration for 48 h led to substantial DNA damage, marked by a significant elongation of comet tails [Figure 5]. These findings are supported by previous studies in different cancer cells.[30,32]
Apoptosis can be triggered through either the extrinsic or intrinsic pathways, both of which require the activation of caspase proteins. Quercetin upregulated the effector caspase 3, which is crucial for poly (ADP-ribose) polymerase (PARP) cleavage and DNA fragmentation and is responsible for producing apoptotic indicators. It is responsible for DNA fragmentation and the morphological changes associated with cell death. The increased activity of caspase 3 in quercetin-treated HeLa cells [Figure 6] further supports the activation of the caspase cascade and the instigation of apoptosis. From the results, it may be postulated that the activation of Caspase-3 and the presence of flavonoids in quercetin, the free radical quenchers, would have promoted DNA damage, resulting in mutations promoting apoptosis of HeLa cells.
Analyzing the binding interactions between ligands and apoptotic proteins is essential for developing new preventive and therapeutic drugs. Intermolecular flexible docking simulations were carried out in our previous study to predict the binding mode (Quercetin ligand) and binding site (apoptotic proteins) and provide insights into how quercetin may influence pro-apoptotic pathways in cells.[33]
Consequently, quercetin may potentially promote apoptosis by directly activating pro-apoptotic pathways or stabilizing pro-apoptotic proteins, thus inducing programmed cell death in cancer cells. This property makes quercetin a promising candidate for anticancer therapies, as it may help to trigger apoptosis in cancerous cells. As quercetin also follows Lipinski’s Rules of Five, it is an ideal candidate for anticancer treatments as it may induce apoptosis in cancerous cells.[34]
While HeLa cells are a well-established model for studying cervical cancer, they represent only one aspect of the disease’s complexity. Future investigations using other cervical and non-cervical cancer cell lines are essential to validate the generalizability of quercetin’s anti-cancer effects and its potential applicability across different cancer types. A key limitation of this study is the lack of in vivo validation, restricting the translation of the promising in vitro results to clinical applications. Future studies should include animal models to evaluate therapeutic efficacy and safety comprehensively. In addition, exploring the synergistic potential of quercetin with other drugs or natural products may pave the way for innovative cancer treatments.
CONCLUSION
Quercetin demonstrates significant potential as an anticancer agent, as evidenced by its ability to inhibit cell growth, induce cell cycle arrest, cause DNA damage, and activate apoptosis in cervical cancer (HeLa) cells. Our previous in silico docking studies further corroborate its efficacy, revealing strong interactions with key proapoptotic proteins. A significantly lower IC50 concentration (25.5 μM) at 48 h compared to 24 h highlights the time-dependent nature of quercetin’s cytotoxic effects. This suggests that prolonged exposure may enhance its potency, providing valuable insights into its potential as a therapeutic agent for cancer treatment over extended periods. In addition, quercetin’s compliance with Lipinski’s rule of five underscores its suitability as a viable therapeutic drug. The study also emphasizes the critical role of caspase-3 activation in quercetin-induced apoptosis, contributing to the growing body of evidence supporting caspase-mediated apoptosis as an effective therapeutic strategy.
Acknowledgment
The authors extend their appreciation to the Researchers Supporting Project number (RSPD2025R966), King Saud University, Riyadh, Saudi Arabia.
Authors’ contributions
SF, SK, and NJ: Conception and design of the study; MB and RS: Methodology and Investigation; RA: Data curation; SK and NJ: Writing - original draft; NP and PS: Writing - review & editing. All authors revised and approved the final version of the manuscript.
Ethics approval
Institutional Review Board approval is not required as the study was performed using established commercial cell lines.
Declaration of patient consent
Patient consent is not required as patient identity is not disclosed or compromised.
Financial support and sponsorship
The work is funded by the Researchers Supporting Project number (RSPD2025R966), King Saud University, Riyadh, Saudi Arabia.
Conflicts of interest
There are no conflicts of interest.
Availability of data and material
The data and materials used in this study will be made available by the corresponding authors upon reasonable request.
References
- Global inequalities in cervical cancer incidence and mortality are linked to deprivation, low socioeconomic status, and human development. Int J MCH AIDS. 2012;1:17-30.
- [CrossRef] [PubMed] [Google Scholar]
- Awareness of cervical cancer among women in Malaysia. Int J Health Sci. 2018;12:42-8.
- [Google Scholar]
- Distribution of human papilloma virus infections of uterine cervix among women of reproductive age-A Cross-sectional hospital-based study from North East India. Asian Pac J Cancer Prev. 2015;16:1519-23.
- [CrossRef] [PubMed] [Google Scholar]
- Human papillomavirus genomics: Understanding carcinogenicity. Tumour Virus Res. 2023;15:200258.
- [CrossRef] [PubMed] [Google Scholar]
- Mycochemistry, antioxidant content, and antioxidant potentiality of the ethanolic extract of Pleurotus florida and its anti-cancerous effect on hela cancer cell line, and antitumor effect on HeLa-implanted mice. Int J Health Sci. 2023;17:18-35.
- [Google Scholar]
- Liposome as nanocarriers for the delivery of Phytomedicines: implication in the treatment of cancer. Int J Health Sci. 2023;17:1-2.
- [Google Scholar]
- The Synergistic Effect of Oxaliplatin and punicalagin on colon cancer cells caco-2 death. Int J Health Sci. 2024;18:33-7.
- [Google Scholar]
- Phytochemical profiling, antioxidant potential and cytotoxic activity of Sargassum prismaticum Extracts: implications for therapeutic applications. Nat Prod Res. 2024;9:1-7. [Epub ahead of print]
- [CrossRef] [Google Scholar]
- Elucidation of the anticancer mechanism of durian fruit (Durio zibethinus) pulp extract in human leukemia (HL-60) cancer cells. Nutrients. 2023;15:2417.
- [CrossRef] [PubMed] [Google Scholar]
- Cancer-preventive activity of Argemone mexicana linn leaves and its effect on TNF-α and NF-κB signalling. Cancers. 2023;15:5654.
- [CrossRef] [PubMed] [Google Scholar]
- Resveratrol as an anti-cancer agent: A review. Crit Rev Food Sci Nutr. 2018;58:1428-47.
- [CrossRef] [PubMed] [Google Scholar]
- Phytochemical profiling, anticancer and apoptotic activity of graviola (Annona muricata) fruit extract against human hepatocellular carcinoma (HepG-2) cells. Int J Zool Appl Biosci. 2020;5:32-47.
- [Google Scholar]
- Phytochemical evaluation and anticancer activity of rambutan (Nephelium lappaceum) fruit endocarp extracts against human hepatocellular carcinoma (HepG-2) cells. Saudi J Biol Sci. 2021;28:1816-25.
- [CrossRef] [PubMed] [Google Scholar]
- A critical review on quercetin bioflavonoid and its derivatives: Scope, synthesis, and biological applications with future prospects. Arab J Chem. 2023;16:104881.
- [CrossRef] [Google Scholar]
- The relationship between phytoestrogen-rich supplements and breast cancer: A multicenter case-control study in Saudi Arabia. Int J Health Sci. 2024;18:35-42.
- [Google Scholar]
- Quercetin, a flavonoid with great pharmacological capacity. Molecules. 2024;29:1000.
- [CrossRef] [PubMed] [Google Scholar]
- Quercetin supplementation and upper respiratory tract infection: A randomized community clinical trial. Pharmacol Res. 2010;62:237-42.
- [CrossRef] [PubMed] [Google Scholar]
- Benefits of quercetin on glycated hemoglobin, blood pressure, PiKo-6 readings, night-time sleep, anxiety, and quality of life in patients with type 2 diabetes mellitus: A randomized controlled trial. J Clin Med. 2024;13:3504.
- [CrossRef] [PubMed] [Google Scholar]
- Anticancer potential of quercetin: a comprehensive review. Phytother Res. 2018;32:2109-30.
- [CrossRef] [PubMed] [Google Scholar]
- Intakes of selected nutrients, foods, and phytochemicals and prostate cancer risk in Western New York. Nutr Cancer. 2005;53:33-41.
- [CrossRef] [PubMed] [Google Scholar]
- Phase i clinical trial of the flavonoid quercetin: Pharmacokinetics and evidence for in vivo tyrosine kinase inhibition. Clin Cancer Res. 1996;2:659-68.
- [Google Scholar]
- Potential ameliorative effect of dietary quercetin against lead-induced oxidative stress, biochemical changes, and apoptosis in laying japanese quails. Ecotoxicol Environ Saf. 2022;231:113200.
- [CrossRef] [PubMed] [Google Scholar]
- Protective effects of quercetin on UVB irradiation-induced cytotoxicity through ROS clearance in keratinocyte cells. Oncol Rep. 2017;37:209-18.
- [CrossRef] [PubMed] [Google Scholar]
- Pharmacological basis and new insights of quercetin action in respect to its anti-cancer effects. Biomed Pharmacother. 2020;121:109604.
- [CrossRef] [PubMed] [Google Scholar]
- Quercetin induces cytochrome-c release and ros by causing G2/M arrest and inducing apoptosis. Food Chem Toxicol. 2013;46:2042-53.
- [CrossRef] [PubMed] [Google Scholar]
- Quercetin modulates signaling pathways and induces apoptosis in cervical cancer cells. Biosci Rep. 2019;39:BSR20190720.
- [CrossRef] [PubMed] [Google Scholar]
- Targets involved in the anti-cancer activity of quercetin in breast, colorectal and liver neoplasms. Int J Mol Sci. 2023;24:2952.
- [CrossRef] [PubMed] [Google Scholar]
- Induction of cell cycle arrest and apoptosis in human breast cancer cells by quercetin. Int J Oncol. 2001;19:837-44.
- [CrossRef] [PubMed] [Google Scholar]
- Flavones and flavonols exert cytotoxic effects on a human oesophageal adenocarcinoma cell line (OE33) by causing G2/M arrest and inducing apoptosis. Food Chem Toxicol. 2008;46:2042-53.
- [CrossRef] [PubMed] [Google Scholar]
- Chemotherapeutic potential of quercetin on human bladder cancer cells. J Environ Sci Health A Tox Hazard Subst Environ Eng. 2016;51:776-81.
- [CrossRef] [PubMed] [Google Scholar]
- Quercetin, a natural flavonoid interacts with dna, arrests cell cycle and causes tumor regression by activating mitochondrial pathway of apoptosis. Sci Rep. 2016;6:24049.
- [CrossRef] [PubMed] [Google Scholar]
- Effects of epigallocatechin gallate and quercetin on oxidative damage to cellular DNA. Mutat Res. 2000;459:211-8.
- [CrossRef] [PubMed] [Google Scholar]
- In silico docking of quercetin compound against the HeLa cell line proteins. Int J Curr Pharm Res. 2015;7:1-5.
- [Google Scholar]
- Quercetin: A potential polydynamic drug. molecules. 2023;28:8141.
- [CrossRef] [PubMed] [Google Scholar]